Cerebrointestinální osa: fyziologický základ pro pochopení zažívání
Christoph Beglinger1
+ Affiliation
Summary
SOUHRN
Beglinger Ch. Cerebrointestinální osa: fyziologický základ pro pochopení zažívání
Přehled sumarizuje vliv střevních tuků na potlačení chuti k jídlu při regulaci příjmu potravy u lidí se zvláštním důrazem na úlohu cholecystokininu. Současné údaje podporují úlohu střevních tuků, zvláště volných mastných kyselin s dlouhým řetězcem, působením CCK jako fyziologické cesty pocitu sytosti. Regulace tohoto pocitu je však komplexní a nezdá se, že by existovaly nějaké vícečetné kontrolní systémy. Je zajímavé, že nutrienty, např. produkty hydrolýzy tuků v tenkém střevě, stimulují uvolnění sytostních peptidů, jako je CCK nebo PYY, sloužících jako signály sytosti. CCK uvolněný z gastrointestinálního traktu lokálním působením natrávené potravy má různé funkce: Stimuluje kontrakci žlučníku a zevní pankreatickou sekreci, inhibuje vyprazdňování žaludku a potlačuje chu» k jídlu. CCK slouží tedy 1. jako pozitivní zpětný signál ke stimulaci zažívacích procesů a 2. jako negativní zpětný signál limitující množství konzumované potravy během individuálního příjmu potravy.
Klíčová slova: chu» k jídlu - sytost - cholecystokinin - ghrelin - glucagon - žaludeční sekrece - oxyntomodulin.
INTRODUCTION
The survival of man depends upon the drive to find food; by accumulating fat in times of plenty food supply and conserving energy in times of food shortage, our ancestors were able to cope with periods of famine. Today, we are faced with the dangers of food excess and obesity, a disease, which has been classified as epidemic by WHO. The understanding of the regulation of energy homeostasis is therefore of prime importance, if we want to cope with this problem on a rational basis. The area includes different specialties such as neurobiology classical endocrinology, nutrition and metabolism. It is currently one of the most exciting and rapidly advancing areas in medical research; it is also one of the most frustrating. Although numerous advances in the scientific understanding have been made, these have not been translated in the practical treatment of human nutritional disorders. In recent years important advances have been made in our understanding of the peripheral signals that regulate appetite from the gut and adipose tissue, and how these act within the brain. This gut-brain interaction in the context of regulation of appetite is the focus of this review.
Appetite, in particular the role of the gastrointestinal tract in its control, was a subject of lively experimental interest to Pavlov and his contemporaries. By the middle of the last century, however, scientific interest in appetite shifted almost exclusively to the brain. In the last three decades, a new interest in the role of the gut in the regulation of food intake has grown. For most people, the amount and the composition of food eaten vary considerably from one meal to the next and from one day to the next. Emotions, social factors, time of day, convenience, and costs are but a few variables that are not biologically regulated, but nonetheless affect meal-to-meal energy intake. As a consequence, daily energy intake is variable within an individual and not correlated with daily energy expenditure(1-5). Nevertheless, food intake is highly regulated. Despite short-term mismatches, most people match energy intake to energy expenditure with great precision. The new information concerning the different control circuits of energy homeostasis has recently been summarised(6,7).
The decision to begin or to stop eating is certainly derived from complex processes. Many factors have been identified as contributors to the control of eating; in particular, several peptides and hormones acting peripherally and in the central nervous system, and neural feedback loops from the gut which involve activation of a variety of gastrointestinal chemo- and mechanoreceptors as well as hepatic chemoreceptors have been identified(8). The growing number of signalling molecules involved in this regulatory system of energy homeostasis highlights its complexity as well as our understanding of it. Here the focus will be on specific pre-absorptive aspects of this complex system, in particular the role of the gastrointestinal tract in the control of food consumed during individual meals. The major determinant of meal size is the onset of satiety, a biological state induced by signals generated during food ingestion that leads to meal termination.
Satiety information generated during meals is largely conveyed to the hindbrain by means of afferent fibres of the vagus from the upper gastrointestinal tract(7). This information converges in the nucleus tractus solitarius (NTS), an area in the brainstem that integrates sensory information from the gastrointestinal tract as well as taste information from the oral cavity(8). Satiety-induced signals that reach the NTS are initiated by a mechanical or chemical stimulation of the stomach and the small intestine during food ingestion.
INTEGRATED DIGESTIVE PROCESSES TO MEALS
The major function of the different digestive processes is the efficient breakdown of macronutrients in order to optimize their absorption. The stomach acts primarily as a reservoir for food components, but also as a principal organ in the initial stages of mixing and grinding of macronutrients. The role of gastric acid in digestive processes is probably not of major importance, but acid and pepsin prepare the macronutrients for further breakdown.
Gastric acid secretion. Stimulatory and inhibitory pathways both at rest and during meal ingestion control acid secretion; the postprandial response is usually divided into three distinct phases of secretion: cephalic, gastric and intestinal(9-11).
The cephalic phase of acid secretion is activated by thought, taste, smell, sight and swallowing of food(9). Acid responses to cephalic stimulation amount to 50-60% of maximal secretagogue stimulated acid responses in humans(9,12,13). Cephalic phase stimulation of gastrointestinal secretions (acid secretion, exocrine pancreatic secretion) occurs by central stimulation of the vagus nerve and is under cholinergic and peptidergic control(14) . The hormone gastrin plays, however, a physiological role in the cephalic phase secretion; intravenous infusion of a selective gastrin receptor antagonist dramatically reduced the cephalic acid response in healthy subjects suggesting that the peptide is integrated in the neural control circuit(15) . The principle mediator of the gastric phase of acid secretion is the peptide gastrin. Gastric distension and, more important, chemical constituents of food provide the strongest stimulation of gastrin release during the gastric phase of secretion(10,11,15-18) . The intestinal phase of acid secretion is predominantly inhibitory. Fat, acid and hyperosmolar liquids within the intestinal lumen induce inhibition of acid secretion.
Exocrine pancreatic secretion. The major function of exocrine pancreatic secretion is the digestion of macronutrients. Pancreatic digestive enzymes have an obvious role in this process, but pancreatic bicarbonate secretion is thought to be crucial for creating a functional intraluminal milieu for the optimal action of digestive enzymes. As for acid secretion it is useful to conceptualize postprandial pancreatic secretion as being the net result of three phases - cephalic, gastric and intestinal(16,19) . The phases overlap in time due to aboral progression of food through the digestive system and they interact with one another.
The cephalic phase of pancreatic secretion is initiated by the sight and smell of appetizing food. The magnitude of the secretory response ranges from 25-50% of the maximal postprandial pancreatic secretory response(19) . In humans, the pancreatic responses to cephalic stimulation are brief and disappear after cessation(19) of the stimulus. The cephalic phase of both gastric and pancreatic secretions is generally assumed to be primarily mediated by vagal cholinergic pathways. Recently the concept was expanded, as it could be demonstrated that a distinct cephalic phase regulates gastro-pancreatic secretions and antroduodenal motility(19) . The mechanisms involve cholinergic pathways, with partial mediation via peptide release(20-22) . When cephalic stimulation is initiated during late interdigestive phase I and early phase II of duodenal motility, an immediate increase of gastric acid output and pancreatic enzyme secretion is noted. Pancreatic bicarbonate secretion is, however, not stimulated with a cephalic phase stimulus. Again, antroduodenal motility is enhanced in parallel with secretion(21) . The cholinergic antagonist atropine is able to abolish the increase in acid output, in pancreatic enzyme secretion and in antroduodenal motility in response to cephalic stimulation supporting the hypothesis that the cholinergic system is the major regulator of the cephalic phase of these digestive processes(20-22) .
It is difficult to study the gastric phase of exocrine pancreatic secretion. The stomach determines the rate of gastric emptying of food components and thus the pancreatic secretory response by regulating duodenal loads of nutrients. The stomach disperses solid food into small particles, thereby facilitating their digestion(23,24) . In addition, there is some degree of intragastric digestion of dietary proteins by pepsin and of dietary lipids by gastric lipase(25) . The role of gastric lipase in lipid digestion has probably been underestimated in the past; new data reveal that gastric lipase can substantially digest dietary fat(26) . The extend of these digestive processes probably determine the magnitude of intestinal phase stimulation of the pancreas by generating peptides and free fatty acids, which are important luminal stimulants of other processes such as regulation of appetite. Intestinal phase stimulation accounts for the majority of the pancreatic response to a meal, and is therefore predominant in the net postprandial secretory output(27) .
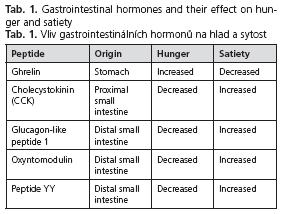
The proximal small intestine is the only site where acid acts to stimulate pancreatic bicarbonate secretion and it is probably the most important site at which digestion products of fat and protein act to stimulate pancreatic enzyme secretion. Long chain fatty acids generated through fat hydrolysis are the most potent stimulants of pancreatic enzyme secretion followed by amino acids and peptides(19) . The mediators of pancreatic enzyme and bicarbonate secretions during intestinal phase stimulation include cholinergic mechanisms and hormones(20-22, 27) . The two classical hormones, secretin and CCK, are two major players in this regulation (Table 1). Long chain fatty acids, proteins and amino acids are the most potent stimulants of CCK release(28,29) , whereas the effect of glucose is minor. The ability of long chain fatty acids to stimulate CCK release is distinctly greater than that of protein or of amino acids.
Is the postprandial release of CCK sufficient to stimulate pancreatic enzyme secretion? Data from our laboratory have provided convincing evidence that endogenous CCK is in important regulator of the intestinal phase of pancreatic enzyme secretion(27,30) . The observation was substantiated in studies using specific CCK receptor antagonists: administration of a CCK receptor antagonist reduced the enzyme response to intestinal food stimulation by ~ 50-60% (19,30) . The fact that specific CCK receptor antagonists can only partially block the stimulatory effect of endogenous CCK supports the existence of additional control mechanisms. Studies performed in patients after vagotomy have provided experimental evidence that the pancreatic enzyme response to intestinal stimulation is reduced by 60%(31,32) . In agreement with this observation, intestinal phase stimulation of pancreatic enzyme output was suppressed by 60-90% when the cholinergic blocker atropine was given without affecting plasma CCK concentrations(33) . The data support the view that pancreatic enzyme secretion is crucially dependent on the cholinergic system with hormones modulating the response.
Acid in the proximal small intestine stimulates exocrine pancreatic secretion, particularly pancreatic bicarbonate(16) . The phenomenon indicates an important role for gastric acid as an intestinal regulator of pancreatic secretion. The mediator of this effect is the hormone secretin. Secretin is released predominantly by acid entering the duodenum. The biologic characterization of human secretin and its release after a meal have provided the experimental basis for this hypothesis(34) . The amount of secretin released after a meal is, however, very small. It has been recognized for a long time that secretin interacts with other pancreatic secretagogues to stimulate pancreatic fluid and bicarbonate output. An interaction between secretin and CCK or between secretin and the cholinergic system has been proven(35,36) .
CONTROL CIRCUITS IN APPETITE AND SATIETY
The decision to begin or to stop eating is certainly derived from complex processes. Many factors have been identified as contributors to the control of eating. A number of recent reviews have summarized the current knowledge of these control circuits; in particular, several peptides and hormones acting peripherally and in the central nervous system, and neural feedback loops from the gut which involve activation of a variety of gastrointestinal chemo- and mechanoreceptors as well as hepatic chemoreceptors have been identified(37-40) . The growing number of signaling molecules involved in this regulatory system of energy ho-meostasis highlights that this process is not only complex but also incompletely understood. In the present review, the focus will be on specific pre-absorptive signals, which are involved in this complex system, in particular the role of the gastrointestinal tract in the control of food consumed during individual meals. The major determinant of the meal size is the onset of satiety, a biological state induced by signals generated during food ingestion that leads to meal termination. Several lines of evidence support the hypothesis that control of the meal size is a component of the feeding response(40) . Satiety information generated during meal intake is largely conveyed to the hindbrain by means of afferent fibres of the vagus(37-40) . This information converges in the nucleus tractus solitarius (NTS), an area in the brainstem that integrates sensory information from the gastrointestinal tract as well as taste information from the oral cavity(37-43) . Satiety-induced signals that read the NTS are initiated by a mechanical or chemical stimulation of the stomach and the small intestine during food ingestion. These signals are triggered by the detection of food molecules by specialised cells (taste receptors) in the mucosa of the small intestine. These cells then release "gut hormones", messenger molecules, which signal to the brain. The hormones (eg CCK, GLP-1, PYY3-36) are believed to work principally by acting on nerve fibres linking directly from gut to brain, termed the vagal afferent pathway. The hormones may also act in a classical endocrine way by traveling in the bloodstream to the brain. These subconscious signals are then integrated by the brain centres, which control food intake, most importantly by areas known as the medulla and hypothalamus(40-43) .
The primary route for transferring information from the gastrointestinal tract to the central nervous system is provided by afferent nerve fibres running in the vagal, splanchnic, and pelvic nerve trunks. In spite of the attention given to the autonomie efferent functions of these nerves, their complement of afferent fibres is far from trivial. Vagal afferent neurons have their bodies in the nodose ganglia, while splanchnic afferents have theirs in the dorsal root ganglia. The afferent nerve fibres are activated by nutrients through chemical changes in the gut lumen and by mechanical deformation of the digestive tube (1-3) . Activation of afferent peptidergic neurons results in the release of peptides at both central and peripheral locations. The role of small intestinal nutrients in activating chemical and mechanical changes in regulating food intake will be discussed below.
GASTROINTESTINAL HORMONES IN APPETITE
The concept that the gut acts as an endocrine organ was developed more than 30 years ago. Several peptides are released from the gut in response to food intake. As shown before, gut hormones influence digestive processes, but they also interact with brain via the gut-brain axis. Evidence for an additional role of in regulating appetite and food intake is emerging. The effects of the major prominent peptides associated with food intake are summarized in Table 1.
Ghrelin. Ghrelin is a 28 amino-acid-long pep-tide found predominantly in the fundus of the stomach, but also in the pituitary gland (44) . Ghrelin is an endogenous ligand for the growth hormone secretagogue receptor (GHS-R). Ghrelin exerts a variety of different biological effects, but the focus has been on its appetite stimulating actions(44-46) ; it has therefore also been labelled as a hunger or orexigenic hormone. Circulating concentrations of ghrelin rise with fasting and are suppressed after eating(47) . Long-chain fatty acids in the small intestine suppress circulating ghrelin levels, whereas medium-chain fatty acids have no effect. Furthermore inhibition of fat hydrolysis in the presence of the lipase inhibitor orlistat abolishes the effect suggesting that the generation of free fatty acids is essential to induce the effect (48) .
The details of the pathways of the orexigenic effects of ghrelin need to be elaborated as ghrelin is released both centrally and peripherally. It is noteworthy that ghrelin or GHS-R knockout mice were found to be normal with respect to food intake or bodyweight.
Cholecystokinin (CCK). CCK was the first gut peptide shown to inhibit food intake(49). It is widely distributed in the mucosa of the upper small intestine and released into the circulation upon stimulation by nutrients (predominantly by fat and amino acids). CCK is a key player in the regulation of digestive processes and its physiological effects include the stimulation of gallbladder contraction and exocrine pancreatic secretion and the regulation of gastric emptying(27-30) .
Administration of CCK reduces food intake, both in animals and in humans(50-55). CCK exerts its effects through the CCK-1 receptors and CCK-1 receptors have been identified on vagal afferent neurons, the principal targets through which CCK induces sensations of satiety. CCK is considered a physiological satiety factor in humans, as pharmacological blockade of the CCK-1 receptor increases appetite and food intake in healthy volunteers(56) . A CCK-1 receptor knockout rat (but not a CCK-1 receptor knockout mouse) is hyperphagic and obese.
Glucagon-like peptide 1 (GLP-1). GLP-1 is a peptide produced by endocrine cells of the distal small intestine as a post-translational product of the glucagon gene upon stimulation by nutrients (predominantly by fat and carbohydrates) (57, 58) . The same cells that secrete GLP-1 produce PYY. GLP-1 is a potent incretin and its main effects include therefore stimulation of insulin secretion and suppression of glucagon, but it exerts also effects on digestive functions (inhibition of gastric acid secretion, retardation of gastric emptying) (57-62) . Administration of GLP-1 reduces food intake, both in animals and in humans(57,63-65) . GLP-1 exerts its effects through specific receptors and these receptors have been identified in neurons of the NTS, the principal targets through which GLP-1 induces sensations of satiety.
Oxyntomodulin. Oxyntomodulin like GLP-1 is a peptide produced by endocrine cells of the distal small intestine as a post-translational product of the glucagon gene upon stimulation by nutrients. The peptide inhibits gastric acid secretion and reduces food intake(66,67) . It exerts its effects through the GLP-1 receptor, although the affinity of oxyntomodulin for this receptor is much lower than that of GLP-1. The precise role of the peptide needs to be established, but it is conceivable the oxyntomodulin and GLP-1 might have different roles.
Peptide YY (PYY). PYY is a member of the PP-fold peptide family(68,69) . The same endocrine cells produce it as GLP-1 upon stimulation by nutrients (mainly digestion products of fat). The major circulating form of PYY is a truncated molecule, PYY3-36, which has a high affinity for the Y2 receptor(70) . Its biological effects include inhibition of gastric acid secretion and inhibition of gastric emptying.
Administration of PYY3-36 reduces food intake, both in animals and in humans(71,72) . As mentioned before, PYY3-36 exerts its effects through the Y2 receptor and as a consequence the peptide does not reduce food intake in Y2 knockout mice. The phenotype of Y2 knockout mice is complex: female PYY knockout mice have an increased bodyweight and fat mass, whereas male knockout mice are resistant to obesity but have a higher fat mass. Controversy exists in the literature on the physiological role of PYY as a satiety factor and the details of the pathways by which the peptide exerts its effects remain to be elucidated(73).
EFFECT OF SMALL INTESTINAL NUTRIENTS ON FOOD INTAKE
As mentioned before, powerful signals arise from the upper gastrointestinal tract during the course of meal intake. These signals include those produced by orosensory stimulation, gastric distension, and most important, by the stimulation of specific chemoreceptors which are triggered by nutrients in the lumen of the small intestine(8,74-76) . The initial sites of action of these peptides are peripheral within the gastrointestinal tract. The peptide-induced satiety messages are then transmitted to the central nervous system by afferent neurons and received in visceral sensory fields of the dorsal hindbrain. The central neural processing required transforming ascending satiety messages into appropriate behaviour (the cessation of eating) and the appropriate sensation (satisfaction) is almost entirely unknown.
The small intestine is a crucial source of satiety signals. In humans, infusion of nutrients (lipids, carbohydrates, protein) into the small intestine is associated with suppression of food intake to a much greater extent than when the same nutrients are given intravenously(77-80) . The interaction of nutrient with specific receptors in the small intestine stimulates the release of satiety hormones such as CCK, GLP-1 or PYY3-36(1,5,8) . All three peptides are able to slow down gastric emptying, thereby prolonging postprandial gastric distension(53,61,71) . Nutrients in the small intestine also delay the transit of food through the small intestine prolonging the time for absorption. Finally, signals from the small intestine are able to modulate the sensations arising from gastric distension after meal ingestion so that they are perceived more as physiologic fullness rather than as discomfort(54) . The last mechanism could be responsible for specific symptoms such as fullness and early satiety perceived by patients with non-ulcer dyspepsia.
Two decades ago it was observed that lipids perfused to small intestine induced a cascade of events related to the regulation of appetite and satiety: Welch et al. (77-80) could show that perfusion of corn oil to the jejunum of healthy volunteers induced early satiety and reduced energy intake; furthermore, jejunal infusions of corn oil decreased hunger feelings. On the basis of their work Welch and co-workers suggested that the effects were caused by CCK release. The work suggested therefore a preabsorptive site of action for the inhibitory effect of fats on short-term food intake in humans. In support of this hypothesis the following compelling evidence is available: 1. intravenous infusion of fat have no effect on food intake(46,49) ; 2. when fats are infused into the small intestine of rats, food intake is reduced within 10 minutes of the start of the infusions, but radiolabeled fat does not appear in the blood until 30 minutes after the start of the infusions. In later studies it was documented that inhibition of fat hydrolysis through the specific lipase inhibitor orlistat abolishes the fat-induced satiety signals from the small intestine(81,82) . In agreement with these observations it was shown that only long-chain fatty acids are able to reduce food intake and stimulate early satiety; medium-chain fatty acids on the other hand have no effect. The mechanisms that mediate the satiating effect of fats in the small intestine initialised by long-chain fatty acids include the release of CCK, GLP-1 and perhaps other hormones(48,83-85) . The action of CCK is mediated through CCK-1 receptors as administration of a specific CCK-1 receptor antagonist completely antagonizes the signal(48) . The results imply that the generation of long-chain free fatty acids through fat hydrolysis is a critical step for fat-induced inhibition of food intake. The signals from the small intestine interact with other regulatory factors controlling food intake and satiety. Nutrients in the small intestine such as long-chain fatty acids interact with gastric distension to regulate appetite(1,5) . Satiety peptides also combine with other signals to influence meal size. For example, when low-dose CCK8 is coupled with a mild gastric distension, meal size is reduced synergistically (55) .
It has become apparent, however, that redundant systems are active. Both CCK and GLP-1 are able to inhibit food intake. CCK-1 receptor or GLP-1 receptor knockout mice do, however, not affect the nutrient status of the animals(50,57) supporting the hypothesis that redundant systems are operative. These systems may replace either peptide when one is absent. In contrast to the results with knock out mice, it has been found that rats lacking CCK-1 receptors are obese and display an altered meal pattern(51,52) . These observations indicate that interactions between different satiety signals are important.
CONSLUSION
During and after eating, hormones are released from endocrine cells of the small intestine in response to nutrients, which modulate digestive functions including appetite. The hormones either modulate vagal activity or affect brainstem function via the vagus or the affect the area postrema activity in an endocrine fashion to reduce appetite, enhance satiety and delay gastric emptying. These hormones include peptides such as CCK, GLP-1, PYY3-36, all released upon stimulation by specific macronu-trients from the small intestine. The peptides have multiple functions, regulating digestive functions but also processes, which contribute to the development of satiety. Satiety can be achieved, at least in part, by inhibition of gastric emptying, and CCK, GLP-1 and PYY have been associated with reducing gastric emptying rates. The level of gastric distension is communicated to the brain by the vagus nerve and both CCK and GLP-1 interact with gastric distension to promote satiety. As illustrated in this review, food intake and eating are at the centre of a complicated system, which regulates digestion and appetite; this system is an integrated gut-brain-energy axis. Neural and hormonal pathways are involved in these processes; the signals are largely triggered in the gut and transmitted to the brain, where they are integrated and processed. Understanding the physiology of these processes is crucial if we want to address the obesity epidemic.
Supported by grant from the Swiss National Science Foundation (Grant No. 320000-118330).
REFERENCES
- 1. Schwartz MW, Woods SC, Porte Jr D, Seeley RJ, Baskin DG. Central nervous system control of food intake. Nature 2000; 404: 661-671.
- 2. Cone RD, Cowley MA, Butler AA, Fan W, Marks DL, Low MJ. The arcuate nucleus as a conduit for diverse signals relevant to energy homeostasis. Int J Obes Relat Metab Disord 2001; 25(Suppl 5): S63-S67.
- 3. Cowley MA, Cone RD, Enriori P, Louiselle I, Williams SM, Evans AE. Electrophysiological actions of peripheral hormones on melanocortin neurons. Ann NY Acad Sci 2003; 994: 175-186.
- 4. Druce MR, Small CJ,. Bloom SR. Minireview: Gut Peptides Regulating Satiety. Endocrinology 2004; 145(6): 2660-2665.
- 5. Strader A, Woods S. Special reports and reviews. Gastrointestinal Hormones and Food Intake. Gastro-enterology2005; 128: 175-191.
- 6. Woods SC, Seeley RJ, Porte D, Jr., Schwartz MW Signals that regulate food intake and energy homeostasis. Science 1998; 280(5368): 1378-1383.
- 7. Woods SC, Schwartz MW, Baskin DG, Seeley RJ. Food intake and the regulation of body weight. Annu Rev Psychol 2000; 51: 255-277.
- 8. Rozengurt N, Wu SV, Chen MC, Huang C, Sternini C, Rozengurt E. Colocalization of the a-subunit of gustducin with PYY and GLP-1 in L cells of human colon. Am J Physiol Gastrointest Liver Physiol 2006; 291: G792-G802.
- 9. Pavlov IP. Beiträge zur Physiologie des Pankreas. Pflugers Arch ges Physiol 1878; 19: 173-189.
- 10. Bayliss WM. Mechanisms of pancreatic secretion. J Physiol 1902; 28: 325-353.
- 11. Feldman M, Richardson CT. Role of thought, sight, smell, and taste of food in the cephalic phase of gastric acid secretion in humans. Gastroenterology 1986; 90(2): 428-433.
- 12. Blair AJ, 3rd, Richardson CT, Walsh JH, Feldman M. Variable contribution of gastrin to gastric acid secretion after a meal in humans. Gastroenterology 1987; 92(4): 944-949.
- 13. Blair AJ, 3rd, Feldman M, Barnett C, Walsh JH, Richardson CT. Detailed comparison of basal and food-stimulated gastric acid secretion rates and serum gastrin concentrations in duodenal ulcer patients and normal subjects. J Clin Invest 1987; 79(2): 582-587.
- 14. Feldman M, Unger RH, Walsh JH. Effect of atropine on plasma gastrin and somatostatin concentrations during sham feeding in man. Regul Pept 1985; 12(4): 345-352.
- 15. Feldman M. Gastric H+ and HC03- secretion in response to sham feeding in humans. Am J Physiol 1985; 248(2 Pt 1): G188-G191.
- 16. Solomon TE. Control of exocrine pancreatic secretion. New York: Raven Press; 1987.
- 17. Beltinger J, Hildebrand P, Drewe J, Christ A, Hlobil K, Ritz M, et al. Effects of spiroglumide, a gastrin receptor antagonist, on acid secretion in humans. Eur J Clin Invest 1999; 29(2): 153-159.
- 18. Blair AJ, 3rd, Richardson CT, Vasko M, Walsh JH, Feldman M. Comparison of acid secretory responsiveness to gastrin heptadecapeptide and of gastrin heptadecapeptide pharmacokinetics in duodenal ulcer patients and normal subjects. J Clin Invest 1986; 78(3): 779-783.
- 19. Adler G, Nelson DK, Katschinski M, Beglinger C. Neurohormonal control of human pancreatic exocrine secretion. Pancreas 1995; 10(1): 1-13.
- 20. Adler G, Beglinger C, Braun U, Reinshagen M, Koop I, Schafmayer A, et al. Interaction of the cholinergic system and cholecystokinin in the regulation of endogenous and exogenous stimulation of pancreatic secretion in humans. Gastroenterology 1991; 100(2): 537-543.
- 21. Adler G, Beglinger C. Hormones as regulators of pancreatic secretion in man. Eur J Clin Invest 1990; 20 Suppl 1: S27-S32.
- 22. Adler G, Beglinger C, Braun U, Reinshagen M, Koop I, Schafmayer A. Cholecystokinin is a regulator of intestinal phase-stimulated PP release. Regul Pept 1990; 30(2): 105-111.
- 23. Meyer JH. Gastric emptying of ordinary food: effect of antrum on particle size. Am J Physiol 1980; 239(3): G133-G135.
- 24. Mayer EA. The Physiology of Gastric Storage and Emptying. In: Physiology of the Gastrointestinal Tract. New York: Raven Press 1994; 929-976.
- 25. Meyer JH, Mayer EA, Jehn D, Gu Y, Fink AS, Fried M. Gastric processing and emptying of fat. Gastroenterology 1986; 90(5 Pt 1): 1176-1187.
- 26. Carriere F, Renou C, Ransac S, Lopez V, De Caro J, Ferrato F, et al. Inhibition of gastrointestinal lipolysis by Orlistat during digestion of test meals in healthy volunteers. Am J Physiol Gastrointest Liver Physiol 2001; 281(1): G16-28.
- 27. Hildebrand P, Beglinger C, Gyr K, Jansen JB, Rovati LC, Zuercher M, et al. Effects of a cholecystokinin receptor antagonist on intestinal phase of pancreatic and biliary responses in man. J Clin Invest 1990; 85(3): 640-646.
- 28. Liddle RA, Goldfine ID, Williams JA. Bioassay of plasma cholecystokinin in rats: effects of food, trypsin inhibitor, and alcohol. Gastroenterology 1984; 87(3): 542
- 29. Liddle RA, Goldfine ID, Rosen MS, Taplitz RA, Williams JA. Cholecystokinin bioactivity in human plasma. Molecular forms, responses to feeding, and relationship to gallbladder contraction. J Clin Invest 1985; 75(4): 1144-1152.
- 30. Beglinger C, Fried M, Whitehouse I, Jansen JB, Lamers CB, Gyr K. Pancreatic enzyme response to a liquid meal and to hormonal stimulation. Correlation with plasma secretin and cholecystokinin levels. J Clin Invest 1985; 75(5): 1471-1476.
- 31. Malagelada JR, Go VL, Summerskill WH. Altered pancreatic and biliary function after vagotomy and pyloroplasty Gastroenterology 1974; 66(1): 22-27.
- 32. MacGregor I, Parent J, Meyer JH. Gastric emptying of liquid meals and pancreatic and biliary secretion after subtotal gastrectomy or truncal vagotomy and pyloroplasty in man. Gastroenterology 1977; 72(2): 195-205.
- 33. Beglinger C, Hildebrand P, Adler G, Werth B, Luo H, Delco F, et al. Postprandial control of gallbladder contraction and exocrine pancreatic secretion in man. Eur J Clin Invest 1992; 22(12): 827-834.
- 34. Christ A, Werth B, Hildebrand P, Gyr K, Stalder GA, Beglinger C. Human secretin. Biologic effects and plasma kinetics in humans. Gastroenterology 1988; 94(2): 311-316.
- 35. You CH, Rominger JM, Chey WY. Effects of atropine on the action and release of secretin in humans. Am J Physiol 1982; 242(6): G608-G611.
- 36. You CH, Rominger JM, Chey WY. Potentiation effect of cholecystokinin-octapeptide on pancreatic bicarbonate secretion stimulated by a physiologic dose of secretin in humans. Gastroenterology 1983; 85(1): 40-45.
- 37. Smith GP. Introduction to the reviews on peptides and the control of food intake and body weight. Neuropeptides 1999; 33(5): 323-328.
- 38. Smith GP. The direct and indirect controls of meal size. Neurosci Biobehav Rev 1996; 20(1): 41-46.
- 39. Smith GP, Gibbs J. Gut peptides and postprandial satiety. Fed Proc 1984; 43(14): 2889-2892.
- 40. Morton GJ, Cummings DE, Baskin DG, Barsh GS & Schwartz MW. Central nervous system control of food intake and body weight. Nature 2006; 443:289-295.
- 41. Berthoud HR. Mind versus metabolism in the control of food intake and energy balance. Physiol Behav 2004; 81: 781-793.
- 42. Moran TH. Gut Peptide Signaling in the Controls of Food Intake. Obesity 2006; 14 (Suppl 1): 250S-253S.
- 43. Cummings DE, Overduin J. Gastrointestinal regulation of food intake. J Clin Invest 2007; 117 (1): 13-23.
- 44. Kojima M, Hosoda H, Matsuo H, Kangawa K. Ghrelin: discovery of the natural endogenous ligand for the growth hormone secretagogue receptor. TRENDS in Endocrinology & Metabolism 2001; 12(3): 118-126.
- 45. Date Y, Kojima M, Hosoda H, Sawaguchi A, Mondal MS, Suganuma T, et al. Ghrelin, a novel growth hormone-releasing acylated peptide, is synthesized in a distinct endocrine cell type in the gastrointestinal tracts of rats and humans. Endocrinology 2000; 141: 4255-4261.
- 46. Cummings DE, Weigle DS, Frayo RS, Breen PA, Ma MK, Dellinger EP, et al. Plasma ghrelin levels after diet-induced weight loss or gastric bypass surgery. N Engl J Med 2002; 346: 1623-1630.
- 47. Wren AM, Small CJ, Ward HL, Murphy KG, Dakin CL, Taheri S, et al. The novel hypothalamic peptide ghrelin stimulates food intake and growth hormone secretion. Endocrinology 2000; 141: 4325-4328.
- 48. Degen LP, Drewe J, Piccoli F, Grani K, Oesch S, Bunea R, et al. Effect of CCK-1 receptor blockade on ghrelin and PYY secretion in men. Am J Physiol Regúl Integr Comp Physiol 2007; 292(4): R1391-1399.
- 49. Smith GP, Gibbs J. Cholecystokinin: a putative satiety signal. Pharmacol Biochem Behav 1975; 3(Suppl 1): 135-138.
- 50. Kopin AS, Mathes WF, McBride EW, Nguyen M, Al-Haider W, Schmitz F, et al. The cholecystokinin-A receptor mediates inhibition of food intake yet is not essential for the maintenance of body weight. J Clin Invest 1999; 103(3): 383-391.
- 51. Schwartz GJ, Whitney A, Skoglund C, Castonguay TW, Moran TH. Decreased responsiveness to dietary fat in Otsuka Long-Evans Tokushima fatty rats lacking CCK-A receptors. Am J Physiol 1999; 277: R1144-R1151.
- 52. Bado A, Durieux C, Moizo L, Roques BP, Lewin MJ. Cholecystokinin-A receptor mediation of food intake in cats. Am J Physiol 1991; 260: R693-R697.
- 53. Moran TH and Kinzig KP. Gastrointestinal satiety signals II. Cholecystokinin. Am J Physiol Gastroin-test Liver Physiol 2004; 286: G183-G188.
- 54. Lai S, McLaughlin J, Barlow J, D'Amato M, Giacovelli G, Varro A, Dockray GJ, Thompson DG. Cholecystokinin pathways modulate sensations induced by gastric distension in humans. Am J Physiol Gastrointest Liver Physiol 2004; 287: G72-G79.
- 55. Gutzwiller JP, Drewe J, Ketterer S, Hildebrand P, Krautheim A, Beglinger C. Interaction between CCK and a preload on reduction of food intake is mediated by CCK-A receptors in humans. Am J Physiol Regul Integr Comp Physiol 2000; 279(1): R189-R195.
- 56. Beglinger C, Degen L, Matzinger D, D'Amato M, Drewe J. Loxiglumide, a CCK-A receptor antagonist, stimulates calorie intake and hunger feelings in humans. Am J Physiol 2001; 280: R1149-R1154.
- 57. Turton MD, O'Shea D, Gunn I, Beak SA, Edwards CM, Meeran K, et al. A role for glucagon-like peptide-1 in the central regulation of feeding [see comments]. Nature 1996; 379(6560): 69-72.
- 58. Hoist JJ. Enteroglucagon. Annu Rev Physiol 1997; 59: 257-271.
- 59. D'Alessio DA, Kahn SE, Leusner CR, Ensinck JW Glucagon-like peptide 1 enhances glucose tolerance both by stimulation of insulin release and by increasing insulin-independent glucose disposal. J Clin Invest 1994; 99: 2263-2266.
- 60. Nauck MA, Weber I, Bach I, Richter S, Orskov C, Hoist JJ, et al. Normalization of fasting glycaemia by intravenous GLP-1 ([7-36 amide] or [7-37]) in type 2 diabetic patients [In Process Citation]. Diabet Med 1998; 15(11): 937-945.
- 61. Nauck MA, Niedereichholz U, Ettler R, Hoist JJ, Orskov C, Ritzel R, et al. Glucagon-like peptide 1 inhibition of gastric emptying outweighs its insulino-tropic effects in healthy humans. Am J Physiol 1997; 273(5 Pt 1): E981-E988.
- 62. D'Alessio D. Peptide hormone regulation of islet cells. Horm Metab Res 1997; 29: 297-300.
- 63. Gutzwiller JP, Drewe J, Goke B, Schmidt H, Rohrer B, Lareida J, et al. Glucagon-like peptide-1 promotes satiety and reduces food intake in patients with diabetes mellitus type 2. Am J Physiol 1999; 276(5 Pt 2): R1541-R1544.
- 64. Gutzwiller JP, Goke B, Drewe J, Hildebrand P, Ketterer S, Handschin D, et al. Glucagon-like peptide-1: a potent regulator of food intake in humans. Gut 1999; 44(1): 81-86.
- 65. Verdich C, Flint A, Gutzwiller JP, Naslund E, Beglinger C, Hellstrom PM, et al. A meta-analysis of the effect of glucagon-like peptide-1 (7-36) amide on ad libitum energy intake in humans. J Clin Endocri-nol Metab 2001; 86(9): 4382-4389.
- 66. Dakin CL, Gunn I, Small CJ, Edwards CM, Hay DL, Smith DM, Ghatei MA, Bloom SR. Oxynto-modulin inhibits food intake in the rat. Endocrinology 2001; 142: 4244-4250.
- 67. Dakin CL, Small CJ, Park AJ, Seth A, Ghatei MA, Bloom SR. Repeated ICV administration of oxynto-modulin causes a greater reduction in body weight gain than in pairfed rats. Am J Physiol Endocrinol Metab 2002; 283: E1173-E1177.
- 68. Adrian TE, Bacarese-Hamilton AJ, Smith HA, Chohan P, Manolas KJ, Bloom SR. Distribution and postprandial release of porcine peptide YY. J Endocrinol 1987; 113: 11-14.
- 69. Taylor RG, Beveridge DJ, Fuller PJ. Expression of ileal glucagon and peptide tyrosine-tyrosine genes. Response to inhibition of polyamine synthesis in the presence of massive small-bowel resection. Biochem J 1992;286:737-741.
- 70. Grandt D, Schimiczek M, Beglinger C, Layer P, Goebell H, Eysselein VE, Reeve JR Jr. Two molecular forms of peptide YY (PYY) are abundant in human blood: characterization of a radioimmunoassay recognizing PYY 1-36 and PYY 3-36. Regul Pept 1994; 51: 151-159.
- 71. Batterham RL, Cowley MA, Small CJ, Herzog H, Cohen MA, Dakin CL, Wren AM, Brynes AE, Low MJ, Ghatei MA, Cone RD, Bloom SR. Gut hormone PYY(3-36) physiologically inhibits food intake. Nature 418: 650-654, 2002. peptide-1 in the central regulation of feeding. Nature 1996; 379: 69-72.
- 72. Batterham RL, Cohen MA, Ellis SM, Le Roux CW, Withers DJ, Frost GS, Ghatei MA, Bloom SR. Inhibition of food intake in obese subjects by peptide YY3-36. N Engl J Med 2003; 349: 941-948.
- 73. Degen L, Oesch S, Casanova M, Graf S, Ketterer S, Drewe J, Beglinger C. Effect of peptide YY(3-36) on food intake in humans. Gastroenterology 2005; 129: 1430-1436.
- 74. Adler E, Hoon MA, Mueller KL, Chandrashekar J, Ryba NJP, Zuker CS. A novel family of mammalian taste receptors. Cell 2000; 100: 693-702.
- 75. Anini Y, Brubaker PL. Muscarinic receptors control glucagon-like peptide 1 secretion by human endocrine L cells. Endocrinology 2003; 144: 3244-3250.
- 76. Dockray GJ. Luminal sensing in the gut: an overview. J Physiol Pharmacol 2003; 54, Suppl 4: 9-17.
- 77. French SJ, Conlon CA, Mutuma ST, Arnold M, Read NW, Meijer G, et al. The effects of intestinal infusion of long-chain fatty acids on food intake in humans. Gastroenterology 2000; 119(4): 943-948.
- 78. Welch I, Saunders K, Read NW. Effect of ileal and intravenous infusions of fat emulsions on feeding and satiety in human volunteers. Gastroenterology 1985; 89(6): 1293-1297.
- 79. Welch IM, Cunningham KM, Read NW. Regulation of gastric emptying by ileal nutrients in humans. Gastroenterology 1988; 94(2): 401-404.
- 80. Welch IM, Sepple CP, Read NW. Comparisons of the effects on satiety and eating behaviour of infusion of lipid into the different regions of the small intestine. Gut 1988; 29(3): 306-311.
- 81. Matzinger D, Degen L, Drewe J, Meuli J, Duebendorfer R, Ruckstuhl N, et al. The role of long chain fatty acids in regulating food intake and cholecystokinin release in humans. Gut 2000; 46(5): 688-693.
- 82. Matzinger D, Gutzwiller JP, Drewe J, Orban A, Engel R, D'Amato M, et al. Inhibition of food intake in response to intestinal lipid is mediated by cholecystokinin in humans. Am J Physiol 1999; 277(6 Pt 2): R1718-R1724.
- 83. Feinle C, Grundy D, Otto B, Fried M. Relationship between increasing duodenal lipid doses, gastric perception, and plasma hormone levels in humans. Am J Physiol Regul Integr Comp Physiol 2000; 278(5): R1217-R1223.
- 84. Feltrin KL, Little TJ, Meyer JH, Horowitz M, Rades T, Wishart J, et al. Effects of lauric acid on upper gut motility, plasm cholecystokinin and peptide YY, and energy intake are load, but not concentration, dependent in humans. J Physiol 2007; 581(Pt 2): 767-777.
- 85. Feltrin KL, Little TJ, Meyer JH, Horowitz M, Smout AJ, Wishart J, et al. Effects of intraduodenal fatty acids on appetite, antropyloroduodenal motility, and plasma CCK and GLP-1 in humans vary with their chain length. Am J Physiol Regul Integr Comp Physiol 2004; 287(3): R524-R533.
To read this article in full, please register for free on this website.
Benefits for subscribers
Benefits for logged users